Urban activities — think construction, transportation, heating, cooling and more — are major sources of greenhouse-gas emissions. Today, a growing number of cities are striving to slash their emission to net zero — here’s what they need to do.
By: Deepa Padmanaban
View the original article here
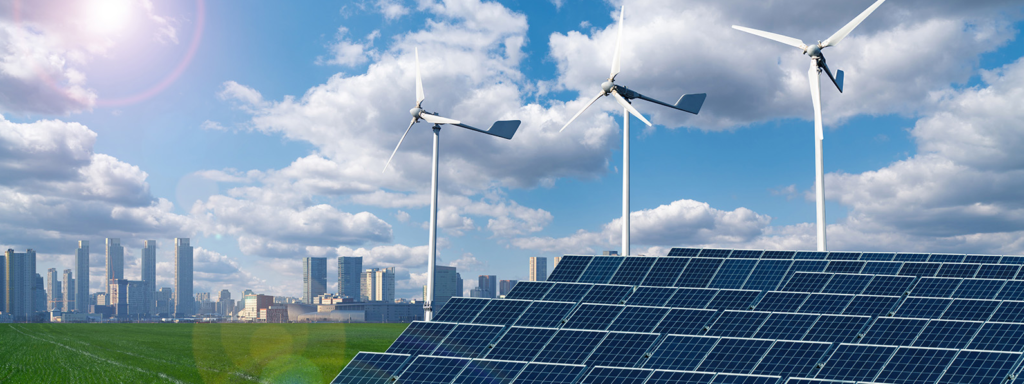
Global temperatures are on the rise — up by 1.1 degrees Celsius since the preindustrial era and expected to continue inching higher — with dire consequences for people and wildlife such as intense floods, cyclones and heat waves. To curb disaster, experts urge restricting temperature rise to 1.5 degrees, which would mean cutting greenhouse gas emissions, by 2050, to net zero — when the amount of greenhouse gases emitted into the atmosphere equals the amount that’s removed.
More than 800 cities around the world, from Mumbai to Denver, have pledged to halve their carbon emissions by 2030 and to reach net zero by 2050. These are crucial contributions, because cities are responsible for 71 percent to 76 percent of global carbon dioxide emissions due to buildings, transportation, heating, cooling and more. And the proportion of people living in cities is projected to increase, such that an estimated 68 percent of the world’s population will be city dwellers by 2050.
“Urban areas play a vital role in climate change mitigation due to the long lifespans of buildings and transportation infrastructures,” write the authors of a 2021 article on net-zero cities in the Annual Review of Environment and Resources. Are cities built densely, or do they sprawl? Do citizens drive everywhere in private cars, or do they use efficient, green public transportation? How do they heat their homes or cook their food? Such factors profoundly affect a city’s carbon emissions, says review coauthor Anu Ramaswami, a professor of civil and environmental engineering and India studies at Princeton University.
Ramaswami has decades of experience in the area of urban infrastructure — buildings, transport, energy, water, waste management and green infrastructure — and has helped cities in the United States, China and India plan for urban sustainability. For cities to get to net zero, she tells Knowable, the changes must touch myriad aspects of city life. This conversation has been edited for length and clarity.
Why are the efforts of cities important? What part do they play in emissions reductions?
Cities are where the majority of the population lives. Also, 90 percent of global GDP (gross domestic product) is generated in urban areas. All the essential infrastructure needed for a human settlement — energy, transport, water, shelter, food, construction materials, green and public spaces, waste management — come together in urban areas.
So there’s an opportunity to transform these systems.
You can think about getting to net zero from a supply-side perspective — using renewable, or green, energy for power supply and transport — which is what I think dominates the conversation. But to get to net zero, you need to also shape the demand, or consumption, side: reduce the demand for energy. But we haven’t done enough research to understand what policies and urban designs help reduce demand in cities. Most national plans focus largely on the supply side.
You also need to devise ways to create carbon sinks: that is, remove carbon from the atmosphere to help offset the greenhouse gas emissions from burning fossil fuels.
These three — renewable energy supply, demand reduction through efficient urban design and lifestyle changes, and carbon sinks — are the broad strategies to get to net zero.

How can a city tackle demand?
Reducing demand for energy can be through efficiency — using less energy for the same services. This can be done through better land-use planning, and through behavior and lifestyle changes.
Transportation is a great example. So much energy is spent in moving people, and most of that personal mobility happens in cities. But better urban planning can reduce vehicle travel substantially. Mitigating sprawl is one of the biggest ways to reduce demand for travel and thus reduce travel emissions. In India, for example, Ahmedabad has planned better to reduce urban sprawl, compared to Bangalore, where sprawl is huge.
Well-designed, dynamic ride sharing, like the Uber and Lyft pools in the US, can reduce total vehicle miles by 20 or 30 percent, but you need the right policies to prevent empty vehicles from driving around and waiting to pick up people, which can actually increase travel. These are big reductions on the demand side. And then you add public transit and walkable neighborhoods.
Electrification of transportation — the supply side — is important. But if you only think about vehicle electrification, you’re missing the opportunity of efficiency.
Your review talks about the need to move to electric heating and cooking. Why is that important?
There’s a lot of emphasis on increasing efficiency of devices and systems to reduce these big sources of energy use, and thus emissions — heating, transport and cooking. But to get to net zero, you also have to change the way you provide heating, transport and cooking. And in most cities, heating and cooking involve the direct use of fossil fuels.
For example, house heating is a big thing in cold climates. Right now, we use natural gas or fuel oil for heating in the US, which is a problem because they are fossil fuels that release greenhouse gases when they are burned. With many electric utilities pledging to reduce the emissions form power generation to near-zero, cities could electrify heating so that the heating system is free of greenhouse gas emissions.
Cooking is another one. Some cities in the US, like New York City and others in California, have adopted policies that restrict natural gas infrastructure for cooking in new public buildings and neighborhood developments, thereby promoting electric cooking. Electrifying cooking enables it to be carbon-emissions-free if the source of the electricity is net zero-emitting.
Many strategies require behavior change from citizens and public and private sectors — such as moving from gasoline-powered vehicles to lower-emission vehicles and public transport. How can cities encourage such behaviors?
Cities can offer free parking for electric vehicles. For venues that are very popular, they’ll offer electric vehicle charging, and parking right up front. But more than private vehicles, cities have leverage on public vehicles and taxi fleets. Many cities are focusing on changing their buses to electric. In Australia, Canberra is on track to convert their entire public transit fleet to electric buses. That makes people aware, because the lack of noise and lack of pollution is very noticeable, and beneficial.
The Indian government is also offering subsidies for electric scooters. And some cities across the world are allowing green taxis to go to the head of the line. Another incentive is subsidies: The US was offering tax credits for buying electric cars, for example, and some companies subsidize car-pooling, walking or transit. At Princeton, if I don’t drive to campus, I get some money back.
The main thing is to reduce private motorized mobility, get buses to be electric and nudge people into active mobility — walking, biking — or public transit.

How well are cities tackling the move to net zero?
Cities are making plans in readiness. In New York City, as I mentioned, newly built public housing will have electric cooking and many cities in California have adopted similar policies for electric cooking.
In terms of mobility, California has among the world’s largest electric vehicle ownership. In India, Ola, a cab company similar to Uber, has made a pledge to electrify its fleet. The Indian government has set targets for electrifying its vehicle sector, but then cities have to think about where to put charging stations.
A lot of cities have been doing low carbon transitions, with mixed success. Low carbon means reducing carbon by 10 to 20 percent. Most of them focus entirely on efficiency and energy conservation and will rely on the grid decarbonizing, but that’s just not fast enough to get you to net zero by 2050. I showed in one of my papers that even in the best case, cities would reduce carbon emissions by about 1 percent per year. Which isn’t bad, but in 45 years, you get about a 45 percent reduction, and you need 80-plus percent to get to net zero. That means eliminating gas/fossil fuel use in mobility, heating and cooking, and creating construction materials that either do not emit carbon during manufacturing or might even absorb or store carbon.
That’s the systemic change that is going to contribute to getting to net zero, which we define in our Annual Review of Environment and Resources paper as at least 80 percent reduction. The remaining 20 percent could be saved through strategies to capture and store carbon dioxide from the air, such as through tree-planting, although the long-term persistence of the trees is highly uncertain.
Are there notable case studies of cities you could discuss?
Denver has been covering the most sectors. Some cities cover only transportation and energy use in buildings, but Denver really quantified additional sectors. They even measured the energy that goes into creating construction materials, which is another thing the net zero community needs to think about. Net zero is not only about what goes on inside your city. It is also about the carbon embodied in materials that you bring into your city and what you export from your city.
Denver was keeping track of how much cement was being used, how much carbon dioxide was needed to produce that cement, called embodied carbon; what emissions were coming from cars, trucks, SUVs and energy use in buildings. They measured all of this before they did any interventions.

The city has also done a great job of transitioning from low-carbon goals (for example, a 10 percent reduction in a five-year span) to deep decarbonization goals of reducing emissions by 80 percent by 2050. During their first phase of low-carbon planning back in 2010, they counted the impact of various actions in each of these sectors to reduce greenhouse gas emissions by 10 percent below 1990 baselines, through building efficiency measures, energy efficiency and promotion of transit, and were successful in meeting their early goals.
Denver is also a very good example of how to keep track of interventions and show that it met its goals. If the city did an energy efficiency campaign, it kept track of how many houses were reached, and what sort of mitigation happened as a result.
But they realized that they’re never going to get down to net zero because, while efficiency and conservation reduce gas use for heating and gasoline use for travel, it cannot get them to be zero. So in 2018, they decided that they’re now going to do more systemic changes to try to reduce emissions by 80 percent by 2050, and monitor them the same way. This includes systemic shifts to heating via electric heat pumps and shifting to electric cars as the electric grid also decarbonizes.
So it’s counting activities again: How many electric vehicles are there? How many heat pumps are you putting into the houses that can be driven by electricity rather than by burning gas? How many people adopt these measures? What’s the impact of adoption?
What you’re saying is that this accounting before and after an intervention is put in place is very important. Is it very challenging for cities to do this kind of accounting?
It’s like an institutional habit — like going to the doctor for a checkup every two years or something. Someone in the city has to be charged with doing the counting, and so many times, I think it just falls off the radar. That was what was nice about Denver — and we worked with them, gave them a spreadsheet to track all these activities.
Though very few cities have done before and after, Denver is not the only one. There are 15 other cities showcased by ICLEI, an organization that works with cities to transition to green energy.
I have worked with ICLEI-USA to develop protocols on how to report and measure carbon emissions. One of the key questions is: What sectors are we tracking and decarbonizing? As I mentioned at the start, most cities agree with tackling energy use in transportation and building operations, and greenhouse emissions from waste management and wastewater. ICLEI has been a leader in developing accounting protocols, but cities and researchers are realizing that cities can do more to address construction materials — for example, influencing choice between cement and timber, which may even store carbon in cities over the long term.
I serve on ICLEI-USA’s advisory committee for updating city carbon emission measurement protocols, and I recommend that cities also consider carbon embodied in construction materials and food, so that they can take action on these sectors as well.
But we don’t have the right tools yet to quantify all the major sectors and all the pathways to net zero that a city can contribute to. That’s the next step in research: ways to quantify all those things, for a city. We are developing those tools in a zero-carbon calculator for cities.